I have been working on materials discovery through the tried-and-true practice of hands-on materials chemistry for over half a century. This is a tradition that has a long history of providing materials that matter for all known products, processes, and devices through the ages. While it is true that most materials have been discovered by serial synthetic methods, sometimes with a little help from serendipity and occasionally accelerated by combinatorial materials chemistry library searches, nevertheless the approach has given civil society most of the materials they need for “better living through materials chemistry”. Yet we continue the search for better and better materials?
Combinatorial materials chemistry did not really disrupt the way traditional materials chemists think and operate, and seems not to have produced anything truly technologically significant, to the best of my knowledge. Today I would contend many practicing materials chemists are trying to figure out whether the emergence of artificial intelligence, machine learning, and robotic automation in the field of materials discovery can really take-off where combinatorial materials chemistry left-off, and disrupt (in a positive way) the field of materials chemistry as we know it today.
As a step in this direction, I have tasked myself with trying to understand how to work at the interface of humans and machines to discover new and better materials. I am actively searching for synergies that can be exploited between current human intelligence, experiential learning and experimental skills practiced by materials chemists and the computational software and hardware that underpins emerging artificial intelligence, machine learning, and robotic automation systems.
Some thoughts skirting around this inquiry can be found in a couple of our recent Advance Science News articles and a paper in the journal Chem: Autonomous chemical synthesis, Prof-bot the autonomous chemistry professor, Catalyst: New Materials Discovery: Machine-Enhanced Human Creativity.
In this opinion editorial, “energy materials discovery” is used as a training ground to explore this question and compare and contrast the old way with the new way of operating in an attempt to discover how humans and machines can work harmoniously together to the benefit of each.
Energy Fact
In 2018, the total worldwide consumption of fossil fuels and renewable forms of energy was 161,248.7984 TWh. This amount of energy is roughly equivalent to 256 soccer fields covered in barrels of oil stacked as high as Mount Everest. It is equivalent to silicon solar panels covering the area of Germany.
Energy Talking Points
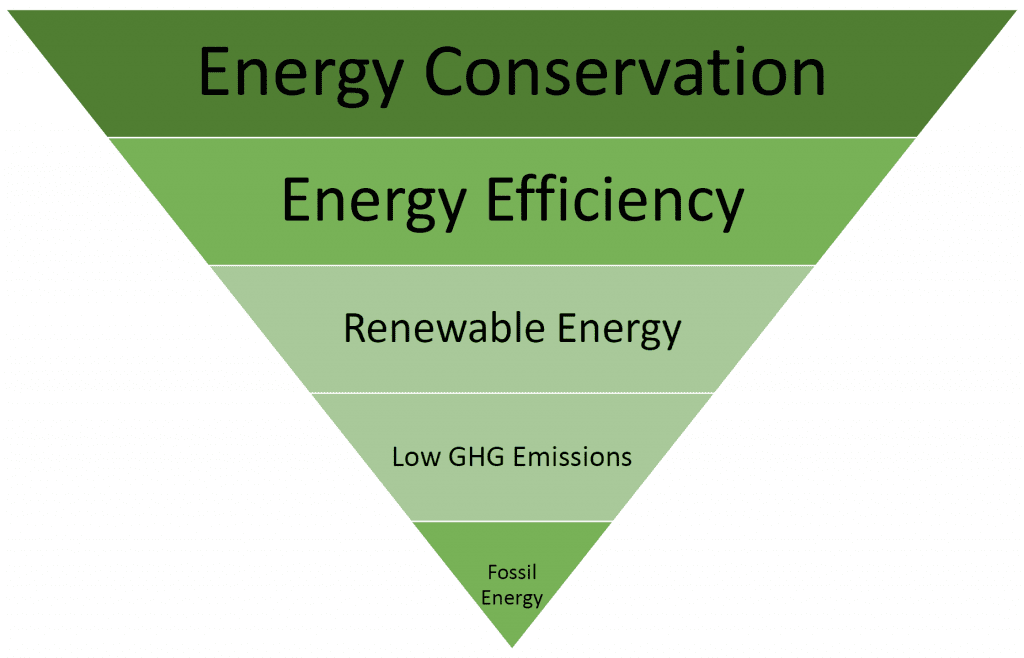
Energy, the capacity to do work, comes in many forms, and while the first law of thermodynamics states it is convertible from one form to another, this is not without losses. Even if we are able in the limiting case to realize an ideal conversion without losses, the second law of thermodynamics further sets the limitation of such a conversion. The ratio of the usable output of an energy system to the input is its energy conversion efficiency, a performance indicator for evaluating and comparing the losses and efficacy of energy systems.
Maximizing energy efficiency translates into minimizing the energy to provide goods and services, which means reduced energy costs for producers and savings for consumers, providing the cost of saved energy compensates for the cost of efficiency enhancements of the energy technology.
When energy was cheap and its environmental impact was low, energy efficiency was not high on the agenda however, with the rise in greenhouse gas emissions and the looming threat of climate change this has become a priority. Projections indicate that improvements in the energy efficiency of transportation, industrial processes, and buildings could reduce around 33% of global energy demands by 2050, with concomitant reductions in greenhouse gas emissions, together forming the bedrock of a global sustainable energy policy.
The key for an energy system to be sustainable however is an essentially unlimited supply of resources to produce the energy and the capacity to meet energy demands, as well as the ability to close the material cycle, emphasizing the need for renewable solar and wind among others with recyclable by-products and net-zero emissions.
One way of tracking progress towards achieving a sustainable energy infrastructure is in the form of an energy hierarchy of energy options as illustrated in the scheme. In this hierarchy, conserving energy is the highest priority followed in decreasing levels of importance by enhancing energy efficiency, using renewable energy and minimizing greenhouse gas emissions with the lowest level in the hierarchy, non-renewable fossil resources.
Energy conservation and enhancing energy efficiency are certainly good for the economics, but they are not essential for a sustainable energy infrastructure. One can have an energy-intensive process that has very low efficiency, but as long as the energy is from a renewable source and the material cycle is closed, this process is sustainable. In other words, only two things are necessary for energy sustainability, a renewable energy source and a closed materials cycle
Energy Materials
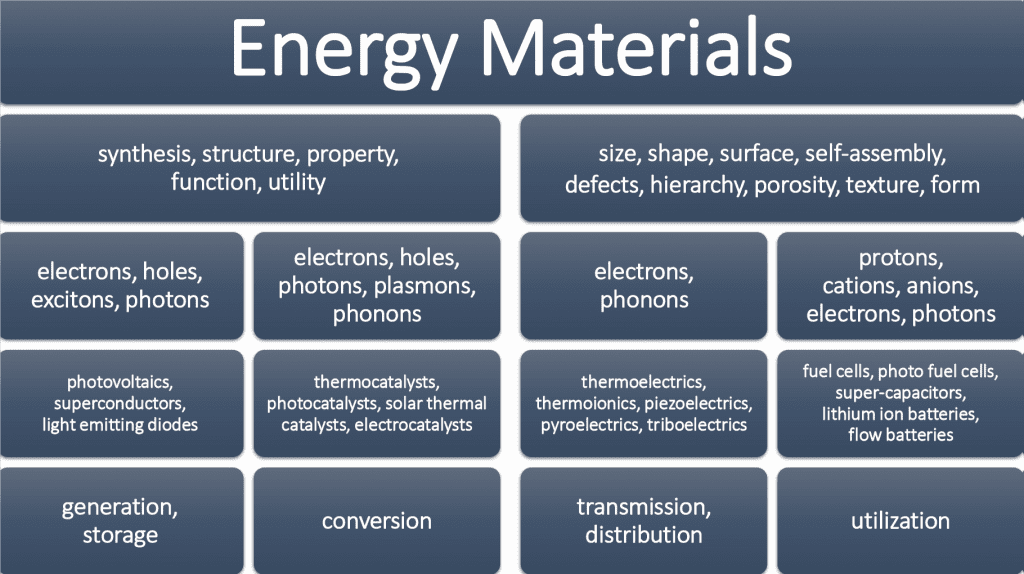
Research and development in the field of energy materials is very broad, spanning the disciplines of materials synthesis, science, and engineering. This endeavor includes determining relationships between structure and properties, learning how they connect to functionality and specific tasks for targeted applications in renewable energy systems, and discovering how to scale, engineer, cost, and recycle materials in particular technologies.
Studying structure-property relationships of energy materials provides information on how to generate energy and transform it from one form to another. This process is exemplified by such conversions as heat to electricity (thermioinics), light to chemical (photocatalysis), chemical to electrical (fuel cells, batteries), mechanical to electrical (piezoelectrics), potential/kinetic to electrical (gravity/wind), electrical to light (light emitting diodes), and light to heat (solar thermal).
Understanding these property-function relationships of energy materials also provides the know-how required for the development of energy storage and retrieval systems, including those based upon reversible chemical to electrical, pressure to electrical, electrical to potential, and thermal to electrical processes, and so forth.
Representative concepts and principles that interrelate the basic science of materials to applications in renewable energy are in the table. Knowledge of this type allows the development of energy transmission and distribution systems for light, electricity, heat and chemicals, required to drive and power transportation, industrial processes, homes, buildings, consumer devices, and the internet.
The discovery of new energy materials or improvement of the properties of ones that exist is key to boosting the efficiency of energy systems, an endeavor geared towards current and future renewable energy technologies. Beyond human intelligence, experiential learning and technical skills, the implementation of artificial intelligence and machine learning augmented by robotic automation offers the potential to accelerate the discovery and optimization of materials that empower renewable energy systems.
One can foresee the integration of tried-and-true materials chemistry approaches, science and engineering and rapid advancements in computerized automation driving a revolutionary shift in the discovery of energy materials, thus heralding a new era of innovative technology development and commercialization to address climate change and global warming.
Energy Systems
The main purpose of an energy system is to generate, convert, store, deliver, and use energy. A synergy exists between energy materials and energy systems; one would not exist without the other. Understanding and exploiting this synergistic relationship underpins the motivation of projects targeting energy materials discovery.
Having targeted an energy material for use in an energy system, the most important task is not just to document all possible contenders but rather to first understand what performance indicators are demanded of the material and the system before meaningful improvements can be undertaken.
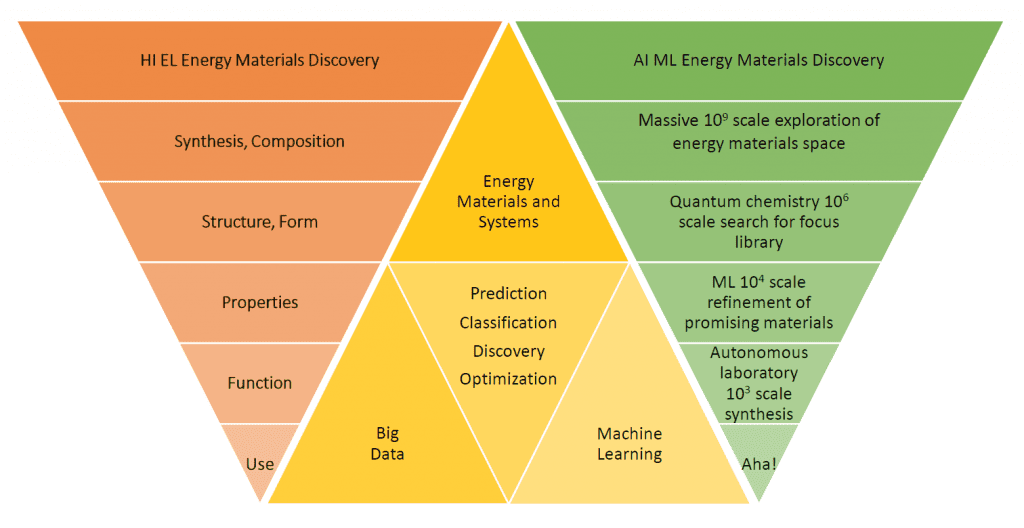
A task is to recognize, understand, and then take advantage of the synergy between human and machine intelligence to accelerate the discovery of superior performing energy materials beyond those that currently exist to deliver smart energy solutions to the energy sector. By working at the human-machine interface, we can use the performance indicators of state-of-the-art energy materials and systems as the springboard for discovering the next generation.
Energy Thought-Tree
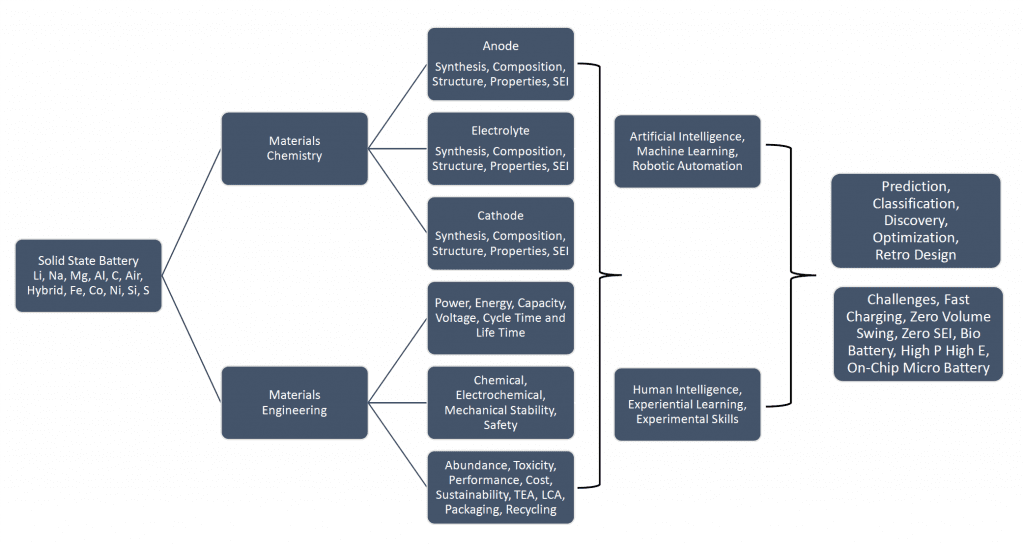
A “thought tree” succinctly illustrates the train of consciousness in the case of an all-solid state inorganic battery, which identifies and depicts, in a rational and ordered form, the connectivity of today’s materials chemistry, science, engineering, and technology questions to what it will take to develop the next generation battery. Is artificial intelligence up to the task?
By understanding how big data and machine learning augmented by robotic automation interact with the existing knowledge base of battery materials and batteries, we can hope to predict, classify, discover, optimize, retro-design and even recycle in the continued development of this and other materials enabled sectors of the economy. But there is more to it than just new materials to enrich the market place.
Implementation Potential of an Energy Material
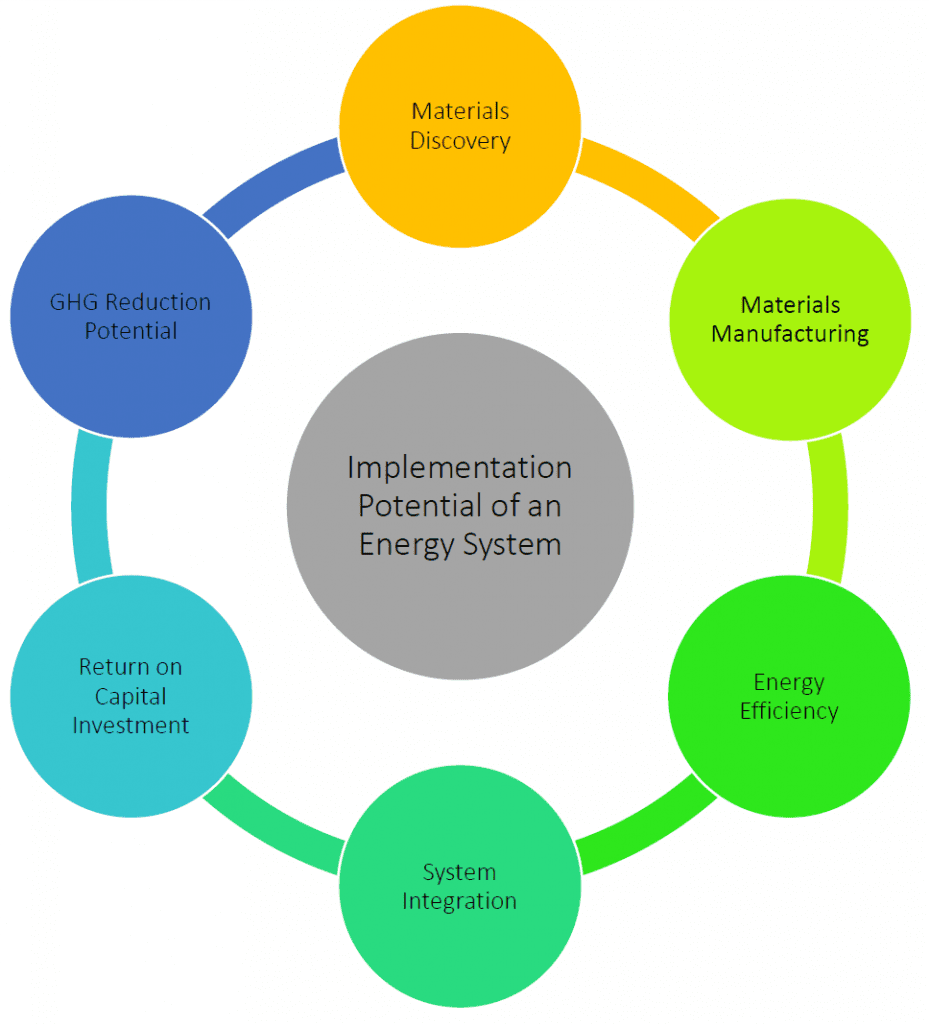
Every energy system ultimately requires a supply of energy. A challenge for research and development of energy systems to become economically feasible has always been to minimize the energy input by maximizing energy efficiency. Often the strategy to achieve these objectives has been through the discovery of new materials or optimization of ones that already exist to improve the performance metrics of existing energy systems. An antithetical argument, however, is that the only motivation for maximizing the energy efficiency is economics. Sustainability does not necessarily requires high energy efficiency. See for example the most important process in nature: photosynthesis, which has an energy efficiency of less than 1%.
In practice there is often more to this than just continuing to make better and better energy materials. It is important to simultaneously critically assess whether improvements in the overall efficiency of an energy system are best achieved through superior energy materials and/or rather system design, the logistics of which are encapsulated in the scheme.
It seems pretty clear from thinking about implementation of a new material into a product, process, or device that many interfaces exist in the “thought-tree” besides that between humans and machines, because ultimately it must compete with existing resources and technologies. A multitude of interdependent factors will influence the outcome of this competition, the relative impact of which need to be appraised. Frequently, life cycle assessments (LCA) and techno economic analyses (TEA) from cradle-to-grave are the tools employed to provide information about bottlenecks to industrialization because in the end the return on capital and operating expenses is a deciding factor for investors expecting a return on their investment in materials discovery.
Post-Script
Blue-Sky Materials Discovery at the Human-Machine Interface
What could possibly be so surprising to this A.I. machine? Simple: The human did something “completely original” that the machine had neither anticipated nor intuited…yet!
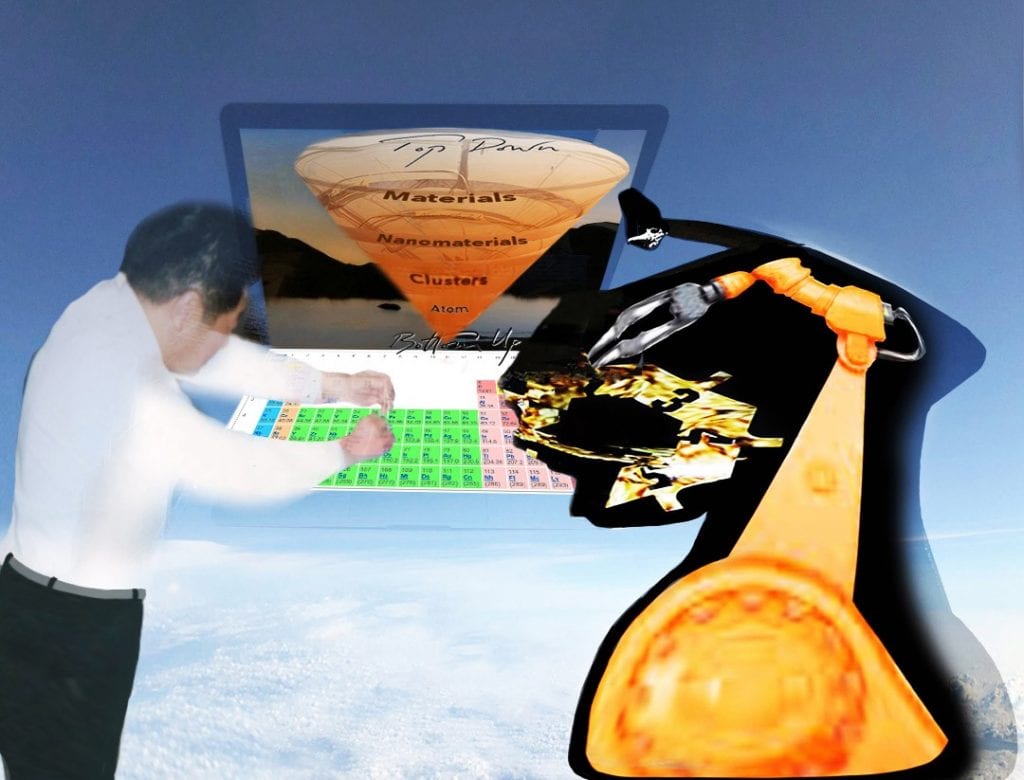
When considering the implementation potential of a new or improved material utilized in various products, processes and devices, we must factor into our equations and symbolic models both human and machine systems: their operations and performances.
More to the point, we need to better understand the whole human/machine interactions and exchanges that invariably determine the optimal “flow” of process and production: from the labs of academia to corporate boardrooms to the businesses that fulfill the demands of customers. This essential flow effect requires a deep understanding of the role leadership plays in orchestrating the entire supply-and-demand chain by the CEOs, CFOs, CIOs and other related stewards of business and industry. Ultimately, this integrated system of human/machine only works effectively and efficiently when the leadership works, although it is also important that there also needs to be an understanding of the desires of the customer/consumer who can reject a new or improved material used in various products and devices despite it being technically superior and more energy efficient and sustainable. There are instances to support this such as a battle for supremacy or adoption of two rival video recording systems between VHS and Betamax. And while it was recognized that Betamax was the superior system technically, for some reason, VHS won the day.
Readers may draw insights and inspiration from these three books: Robert J. Thomas’s “Crucibles of Leadership: How to Learn from Experience to Become a Great Leader“. Foreword by Warren G. Bennis (Harvard Business Review Press, 2008); Warren G. Bennis and Robert J. Thomas’s Geeks & Geezers: How Era, Values, and Defining Moments Shape Leaders. Foreword by David Gergen (Harvard Business School Press Supported by Accenture, 2002); and Robert J. Thomas’s, “What Machines Can’t Do: Politics and Technology in The Industrial Enterprise” (University of California Press, 1994).
Dr. Thomas, an associate partner and senior fellow with the Accenture Institute for Strategic Change, who understands the complexity of managing the innovation process, suggests that we must consider together the visionary thought leaders and operationally brilliant businesses and sophisticated V.I. (Virtually Intelligent) thinking machines, which are now tasked with transforming abstract concepts into concrete realizations.
At the end of the day, our best A.I. and M.L. tools, which we completely rely on to gather data, analyze and interpret it from countless perspectives, will be “required reading” and practice, so to speak. These books, and hundreds of other outstanding literature and resources, must be absorbed in order to “grow” our industries and businesses based on a mutually shared vision of what we can accomplish building more effective materials-based systems for sustaining our One World community.
Finally, to sum up the proposition here, consider the wisdom of the late Dr. Stephan L. Chorover, MIT Professor of Psychology, Brain and Cognitive Sciences, composed in hisworkbook, titled “Homework: An Environmental Literacy Primer” (Collaborative Learning Systems, 1994). Dr. Chorover has written: “[We need to] do something scientifically and pedagogically sound that may help to enhance the chances that those who come after us will be in a position to create a human ecologically sustainable future for themselves and their own descendants … I have gradually come to believe that the prevailing scientific paradigm is insufficient to the task of defining and dealing with our planetary predicament, and that it is going to take a profound rearrangement of human/ecological relations — a mentally and behaviorally broad and deep neurobiological, psychological and sociocultural reorientation — a “paradigm” shift, if you will, for humanity to have a chance of achieving a human ecologically more sustainable future” (II. Paradigms, p.29).
The paradigm shift that Dr. Chorover illuminates and that Dr. Thomas articulates in his book, “What Machines Can’t Do”, is as relevant today as it was twenty-six years ago when the idea of A.I. and advanced thinking machines was still in its infancy.
Written by: Geoffrey Ozin1 and Todd Siler2
1Solar Fuels Group, University of Toronto Ontario, Canada, Email: [email protected], Web sites: www.nanowizard.info, www.solarfuels.utoronto.ca, www.artnanoinnovations.com. 2ArtScience Productions, Denver, Colorado, USA, Email: [email protected], Web Site: www.toddsilerart.com/home