With the advent of flat screens and LEDs, cathode-tube TVs are no longer present in our living rooms. However, this does not mean that photocathodes — namely materials that are able to eject electrons when impinged by light — have disappeared from our lives. This supposedly outdated technology is in fact at the core of future devices such as night-vision goggles for people and self-driving vehicles. Photocathodes are also essential components of electron microscopes, where a beam of accelerated electrons allows scientists to peek into the nanoscale world. Last but not least, extremely focused electron sources are needed for the next-generation of particle accelerators, which are used, among other purposes, to generate ultrashort light pulses (with duration up to a billion times shorter than a billionth of a second!) that will allow us to “see” atoms in materials and follow their dynamics in real time.
On these premises, it is not hard to figure out that efficient materials that are able to emit such ultra-bright and focused electron bunches have become the new Holy Grail for a vast community of scientists. For decades, metals have been used to build photocathodes, due to their high density of charge carriers and the relatively little amount of energy requested to extract them. However, the frequencies of the electrons emitted by metallic surfaces typically lie in the ultra-violet region of the spectrum, which requests an energy-demanding process to convert them to visible frequencies. Moreover, metals get easily heated, which is also a major source of energy dispersion.
For all these reasons, the hunt for alternative materials, which are able to efficiently emit electrons in the visible or infrared range of the spectrum, has engaged numerous groups around the globe. This condition can be fulfilled by certain semiconducting materials and, in particular, by the family of multi-alkali antimonides. This class of crystalline systems is composed of one or more alkali metals (Na, K, Rb, and Cs) bound to antimony atoms (Sb). The presence of alkali metals, hosting only one electron in their outermost atomic shell, ensures relatively low energy for the electrons to be ejected, which is an essential pre-condition for efficient photocathodes. Experimentally, these materials are typically deposited either simultaneously or sequentially on a metallic substrate. This technique, although very effective and convenient, does not enable a straightforward control of the stoichiometry of the sample, nor of its crystal structure. On top of this, the extreme sensitivity of multi-alkali antimonides to atmospheric agents demands ultra-high-vacuum conditions for both growth and for characterization, which has to be performed in situ. All these issues have so far limited the opportunities for in-depth characterization of multi-alkali antimonides, whose preparation recipes have been developed mainly through trial-and-error procedures.
Computational simulations are therefore extremely appealing to identify the most effective material compositions for photocathode applications. In order to do so, it is essential to gain insight into the microscopic mechanisms ruling the emission of electrons. This requires necessarily a quantum-mechanical approach.
To achieve this ambitious goal, the team “High brightness electron beams” at the Helmholtz-Zentrum Berlin and my “Electronic Structure Theory” group at the University of Oldenburg have joined forces for more than two years now. Our collaboration has demonstrated that synergy between experimental synthesis and characterization and quantum-mechanical simulations is key to advance along this research line.
In particular, we have shown that X-ray photoemission spectroscopy is a viable way to connect experimental and theoretical results in determining the correlation between stoichiometry and the efficiency of multi-alkali antimonides in emitting electrons.
Most recently it has been shown that also X-ray absorption spectroscopy can provide additional information to identify the network of chemical bonds, namely the chemical coordination, of specific atomic species.
This has now been demonstrated in my theoretical/computational work focused on caesium-antimonide (Cs3Sb), a representative material of multi-alkali antimonides, and published in physica status solidi (RRL) – Rapid Research Letters. Depending on their specific position inside the crystal, the three Cs atoms in the stoichiometric unit cell of Cs3Sb are characterized by a specific network of atoms to which they are bound. This coordination gives rise to distinct X-ray absorption signals when a given atom is excited at a specific frequency in resonance with the energy of one of its core electrons. Consequently, when the atom itself and/or its local environment is perturbed (for example, by the presence of defects in the crystals) the response to X-ray radiation will correspondingly be altered, thereby providing a hallmark for the identification of the stoichiometry, the chemical composition, and the crystal structure of the material.
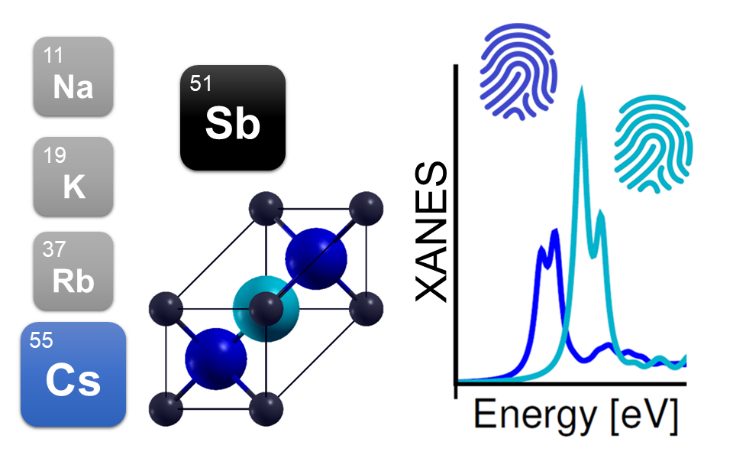
Such an approach was already successfully applied to the case of gallium oxide, a transparent conducting oxide with promising characteristics for the next generation of electronic devices. Also in that case, the same state-of-the-art theoretical/computational approach was employed.
As the subtle spectral features described above are hardly detectable in experiments, a theoretical framework that does not rely on empirical parameters and that yet includes all the necessary ingredient to obtain a quantitative description of the excitation process is needed.
The results of this present study contribute to the advancement in the discovery of novel photocathode materials and in the characterization of their properties, providing a reliable reference for the interpretation of X-ray absorption experiments. Furthermore, it paves the way for further analysis on various composition and stoichiometries of multi-alkali antimonides and related semiconducting materials for the next generation of ultra-bright electron sources.
Reference: C. Cocchi, ‘X‐Ray Absorption Fingerprints from Cs Atoms in Cs3Sb‘, Physica Status Solidi (RRL)–Rapid Research Letters, (2020) DOI: 10.1002/pssr.202000194