Why Do We Care?
Nature is the archetype chemist having synthesized everything ‘under the sun’. Nowhere is this more apparent than in photosynthesis practiced by plants, algae and bacteria that convert sunlight, carbon dioxide and water into stored chemical energy and oxygen and maintains life on earth. Moreover, the chemical capacity of photosynthetic organisms to convert carbon dioxide to organic compounds is around 100 G Tons annually making it the ‘largest chemical factory on earth’.
For a billion years since the emergence of oxygenic photosynthesis on earth the symbiotic cycle of carbon capture, carbon fixation and carbon utilization has remained in balance but in recent times with population growth and increasing demands for food, clean water, air and energy the concentration of carbon dioxide in the troposphere from the combustion of fossil fuels is gradually increasing by about 2 ppm annually, has attained record highs and is continuing to increase. As global consumption increases especially in the developing world, carbon dioxide emissions are expected to keep on rising to levels that potentially threaten life on earth.
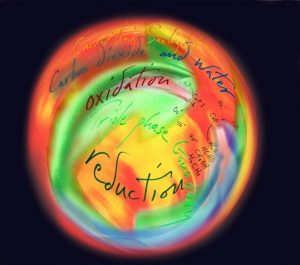
Imagine life in a ‘clean house gas’ rather than a ‘green house gas’ world; this will require a change of human behavior from that practiced in the Anthropocene era where the Earth’s ecosystems have been negatively impacted by humans to the “Sustanocene” age where humans strive to heal the Earth through renewable technologies to make things better. Image: Todd Siler and Geoffrey Ozin – ArtNanoInnovations.
Whether one is a believer or a disbeliever in the greenhouse gas effect, a controversial and divisive issue scientifically, socially, economically and politically, it is surely a moral imperative that decisive preventative action be taken now in order to stabilize the concentration of carbon dioxide in earth’s atmosphere to minimize the risks of the doomsday scenario resulting from global warming, ice-cap and glacier melting, ocean acidification and geopolitical instability.
In the words of Nobel Laureate Jean Marie Lehn, “if it exists it can be synthesized” implying the ability of chemists to make materials that mimic the redox chemistry of photosystem II and photosystem I in photosynthetic plants, algae and bacteria to produce fuel from the sun, carbon dioxide and water is within our grasp.
If this utopian vision of solar fuels from carbon dioxide capture and recycling could be reduced to practice to make greenhouse gas an enabling chemical resource rather than a waste stream and if the process could be conducted at a technologically significant efficiency, at a globally relevant scale and at a cost competitive with fossil fuels, then the development of a sustainable future for following generations becomes an attainable goal.
Solar Fuels Materials Dilemma
The challenge in designing and making materials that can transform carbon dioxide, water and sunlight to an energy rich fuel akin to Nature’s photosynthesis, is the one often faced by scientists and engineers working in the field of advanced materials technology, namely one of providing processes and devices that either perform at high efficiency but utilize critical materials that are in short supply and too pricey or rather work at low efficiency but use materials that are earth abundant and accessible at a competitive cost. So the difficult choice one has to make in today’s solar fuels research is whether or not to focus ones efforts on reducing the cost of a high efficiency process based on rare expensive materials or improving a low efficiency process founded on common cheap materials.
An added requirement for a viable artificial photosynthesis process, which is able to handle the rising concentration of greenhouse gas in the troposphere at a level that can rival natural photosynthesis, necessitates searching for a material that can transform carbon dioxide, water and sunlight to an energy rich fuel with economy of scale and cost of manufacturing. From a practicality standpoint this will likely favor a gas phase light driven heterogeneous photocatalytic process rather than aqueous phase dispersion photocatalysis or aqueous photoelectrochemistry with control over pH and ionic strength and an applied voltage bias to overcome the extra potential required to drive an energetically uphill solar fuel reaction.
Hence one is forced to direct research efforts on a gas phase photoprocess using what tantamount to be ‘zero cost’ photoactive materials with elemental compositions in abundance and lacking toxicity, exemplified by metal oxides, carbon and silicon. These will require shrewd size, shape, surface and interface materials engineering, judicious incorporation of additives and control of defects, and thoughtful design of the way these materials are integrated to an architecture that optimizes the optical, electrical, photophysical and chemical requirements of the system.
All of these considerations are important to avoid future production issues associated with materials scarcity and economy of scale, commonplace requirements for the successful operation of large volume production chemical manufacturing industries.
Can we Match Nature?
Establishing photosynthetic efficiency of plants, algae and bacteria for converting sunlight, carbon dioxide and water to chemical energy is a complex issue that depends on the type of organism and environmental conditions and whether the efficiency is averaged over an annual cycle estimated at 1-3% or growing season at 3-7% (Science, 2011, 332, 805-811). These natural photosynthesis efficiencies are to be compared with the best laboratory mimics researched over the past thirty years which currently are roughly three orders of magnitude less.
The challenge therefore is to discover materials that are able to more efficiently harvest and utilize sunlight, and catalyze the photo-oxidation of water and photo-reduction of carbon dioxide to energy rich fuels such as methane or methanol, illustrated in the scheme. The goal is to synthesize photoactive materials able to chemically couple these light driven redox reactions together and achieve conversion rates and efficiencies of carbon dioxide and water into energy rich fuels at a level that begins to match nature’s photosynthesis.
About 85% of the world’s energy supply is transported in the form of gaseous and liquid, carbon containing fuels!
Why Has Progress Been So Slow?
While water oxidation and carbon dioxide reactions that generate solar fuels look simple on paper in practice they are exceptionally demanding to implement in practice as they involve thermodynamically uphill, multi-electron, multi-hole and multi-proton processes occurring on a multi-component photo-catalyst as envisioned in the scheme. The photo-catalysts needed to facilitate these processes, besides having to be made of earth abundant, non-toxic, light-stable, scalable and low cost materials also have to satisfy additional requirements that include, high surface area and porosity to maximize the adsorption, transport and desorption of reactants, intermediates and products as well as strong visible light absorption to enable the efficient generation, transport and utilization of electrons, holes and protons at water oxidation and carbon dioxide reduction centers. Perhaps the greatest challenge is how to tailor nanomaterials with long-lived charge-separated states required to power multi-electron, multi-hole, multi-proton chemistry that underpins the formation of solar fuels.
In the context of light absorption, a number of optical techniques are potentially useful for boosting the effectiveness by which light is harvested in the photo-active material including:
1. structuring to increase the effective optical path length by multiple light scattering or photonic crystal slow photon amplification
2. surface plasmon resonance to enhance local electric fields and optical absorption strength
3. up-conversion to transform non-absorbed infrared to absorbed visible light
4. multiple-carrier generation to achieve quantum yields greater than 100%
One paradigm in the field of solar fuels is that a single nanostructured material can provide electronic band energies and band gaps that under solar insolation facilitate both water oxidation and carbon dioxide reduction. Another is that these requirements are best achieved in a multi-component heteronanostructure in which electronic band energies and gaps of the constituent materials are matched to the solar spectrum and oxidation and reduction potentials for water and carbon dioxide. The interface between the components of the hetero-nanostructure ideally should be lattice matched and their band energies and gaps tailored to optimize the photo-generation and separation of electron-hole pairs to be competitive with their recombination. In addition their electrical conductivity and diffusion length should be as high as possible to minimize recombination of these electrons and holes and facilitate their transport to surface reaction sites to do productive chemistry.
Equally important is that co-generated protons from water need to be able to rapidly diffuse from the surface of the oxidizing component of the hetero-nanostructure to the reducing one and react with carbon dioxide and electrons to form the carbon-hydrogen bonds of the organic product. This may need the assistance of an organic or inorganic or organic-inorganic hybrid proton conducting material to assist simultaneous proton and electron transport between the oxidizing and reducing components.
Additional considerations involve complications arising from carbon residues formed on the surface of the hetero-nanostructures during their synthesis originating from organics in precursors, ligands and solvents. Adventitious carbon on the surface of the hetero-nanostructure could be hydrophobic and would have the effect of reducing the adsorption of water and carbon dioxide and the absorption of light thereby reducing the efficiency of producing solar fuels.
Other difficulties concern competing photo-oxidation reactions of methane or methanol by co-generated oxygen and formation of hydrogen from recombination of photogenerated protons and electrons from the photo-oxidation of water, both of which can reduce the efficiency of converting carbon dioxide and water to solar fuels.
If a photosynthetic mimic is to really produce solar fuels on a globally significant scale it will have to efficiently and economically capture and cleanup carbon dioxide from thin air and in a chemically and optically engineered gas phase photocatalytic process convert it and water into methane or methanol and separate these organic products from co-generated oxygen, at a cost that is competitive with their production from fossil fuels.
What Have We Learnt?
In all studies reported to date, conversion rates and efficiencies are about three orders of magnitude too low to be of practical significance and the challenge has been to try to understand the reasons for the poor performance and to find materials, chemical and optical engineering remedies for its improvement.
A major impediment in earlier work has been finding reliable means to sort out fact from fiction in the production of solar fuels. The literature since the beginning of the field has been replete with reports that as-synthesized and post-treated materials produce organics at significant rates but only very recently have 13CO2 isotope tracer methods been employed to decide unequivocally whether they originate from carbon dioxide or carbon residues on the surface of the photo-catalysts or both. After much study the consensus is that one of the best ways to reduce adventitious carbon to manageable levels is through ultraviolet light post-treatment of photo-catalysts in humid air or in pH and ionic strength controlled aqueous solution.
Designing and making well-defined nanostructures with all the necessary features mentioned above, to reduce to practice an efficient solar fuels photocatalyst, has proven to be much more challenging than originally anticipated by research scientists and initially expected by government, industrial and private sponsors of this research.
At this point in time a number of key questions still remain to be answered to realize the dream of fuel from the sun at a technologically significant rate, efficiency and scale. These include:
1. how to choose solar fuels nanomaterials
2. how to integrate them into a higher level architecture
3. how to harvest and amplify the absorption of sunlight to optimize the formation, separation and transportation of multiple electrons, holes and protons
4. how to retard the recombination of photo-generated electron-hole charge-separated states and favor the formation of solar fuels
5. how to utilize these photo-generated charge carriers to oxidize water and reduce carbon dioxide to store solar energy in the form of energy rich chemical bonds, at a rate and efficiency that matches or exceeds photosynthesis
6. how to scale fuel from the sun technology to cope with the global emission rate of anthropogenic carbon dioxide at a cost that is economically competitive with fuel from the earth
The ‘super-leaf’ challenge is x1000!
Steps Toward, a Sustainable Future
Approximately 75% of the current 48 Gt CO2 equivalents per year of anthropogenic emissions of greenhouse gases are due to combustion of fossil fuels and until these emissions are reduced to zero, human interference with the climate and its associated social, economic and political uncertainties will continue.
Solar fuels research aimed at the sunlight powered conversion of carbon dioxide and water vapor to methane holds out the promise of large-scale electrical generation fueled entirely by the energy of the sun. One could envision a photo-reactor system would be employed as a ‘bolt on’ technology used in conjunction with existing, well-established energy systems and combustion technologies, minimizing the capital and infrastructure cost of effecting the transition to sun-powered electricity. The result would be to wean electrical generation off fossil fuels and to reduce to zero the CO2 emissions of existing gas-fired electrical generating plants – an enormous step toward creating a sustainable energy future.
Alternatively, the photo-reactor system might be configured to generate methanol – a clean-burning liquid fuel that can readily be stored and transported. This methanol could be used in a wide variety of applications ranging from the very large (e.g. production of industrial chemicals) to the very small (e.g. power generation in homes and businesses). It could also used in internal combustion engines with minimal modifications to energy infrastructure and engine design, enormously reducing reliance on fossil fuels and reducing the contribution of vehicles to global CO2 emissions.
What is needed now are rudimentary materials, chemical and optical engineering analyses of various options imagined for these kinds of solar fuels technologies in order to gain a preliminary understanding of the scientific, technical, economic, and commercialization challenges that different approaches to carbon capture and solar recycling will inevitably face with respect to competing renewable and non-renewable energy sources.
While the ‘super-leaf’ concept is elegant in its simplicity and powerful in terms of its promises for a sustainable future, it will only transition from laboratory curiosity to technological reality if the materials and engineering costs are understood and proven to be competitive with alternative approaches for making methane and methanol.