Electrocatalysts belong to a special class of catalysts that facilitate chemical reactions through the transfer of electrons. Over the past several decades, their applications have extended beyond the laboratory to creating electronic sensors, fuel cells, and batteries, and are poised to aid in the transition to a fossil-fuel-free economy.
While many advancements have been made in designing state-of-the-art electrocatalysts, current catalysts are made from alloys of expensive and rare elements, which not only limits their applicability to industrial applications but also significantly contributes to environmental damage as a result of harmful mining and processing practices. Thus, more cost effective and abundant materials are still needed to make industrial applications of these technologies more feasible. But identifying new allows is not an easy task.
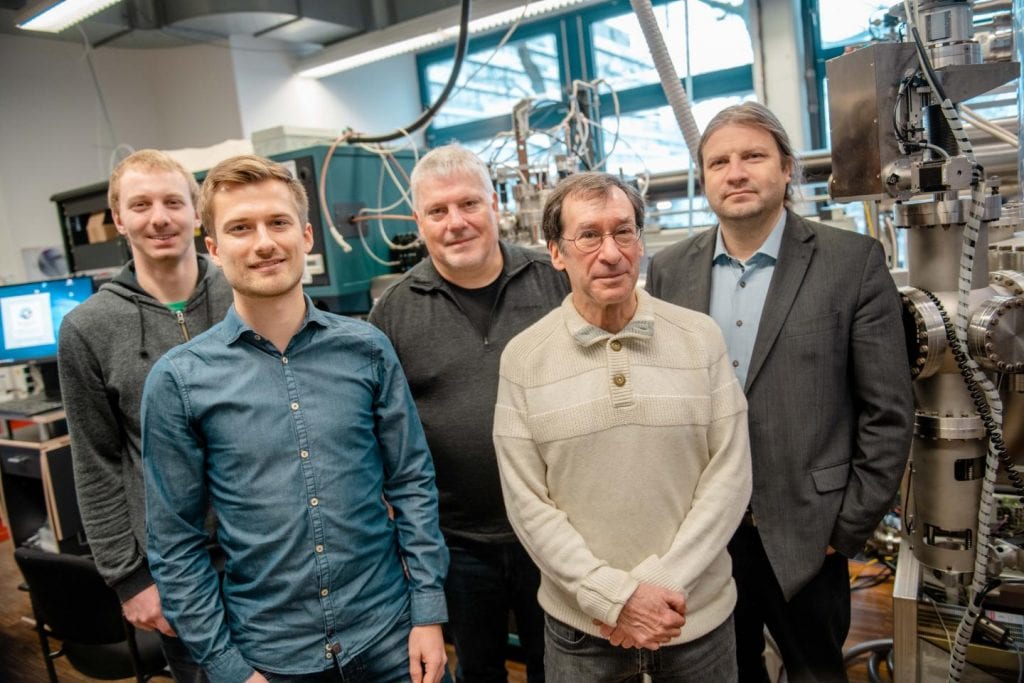
A team of researchers from Ruhr University Bochum, Germany, have proposed that complex solid solutions (also called high entropy alloys) in the form of nanoparticles offers a solution to this challenge.
“The properties of electrocatalysts are generally strongly dependent on the intrinsic properties of the individual elements,” said the authors of the study, Tobias Löffler, Wolfgang Schuhmann, and Alfred Ludwig, in an email. “Specifically, the material dependent binding energy for reactants to the catalyst surface defines the activity and thus the efficiency of the investigated reaction. The formation of a homogeneous distribution of five or more elements within a single solid solution phase activates a completely new interplay of elements, thus breaking with the previously existing rules and inducing a paradigm shift.”
Creating alloys has been extensively used to as a means of enhancing or conferring desirable properties to metals; this usually involves mixing small amounts of what is termed “secondary elements” to a primary element that makes up a large percent of the material’s composition.
Complex solid solutions are alloys that are formed by mixing five or more primary elements together. This strategy has become popular in the last decade or so, yielding materials with exceptional mechanical, physical, and chemical properties that depend not just on the elements that make up the complex solid solution, but how they interact with one another.
“This feature makes this catalyst class very promising with exciting potentials by overcoming existing limitations in element choice and fine tuning of properties,”added the authors. “The complex interaction of many elements enables that new catalysts are not limited by the intrinsic properties of the constituting elements any more, implying that also transition metals can be used, while simultaneously enabling ideal binding energies. Accordingly, many elements can possibly be used for these complex solid solutions.”
Optimization of the catalyst’s elemental composition allows for increasing the number of active sites with favorable adsorption energy for the reaction of interest, and thus, the optimal choice of elements and their respective ratio can generate very active catalysts. “However, the complex interaction between the elements makes it impossible to predict which elemental combinations are potentially promising for which reaction,” said the authors. ” A fundamental understanding of the working principles [of this new class of catalyst] is still very poorly understood. Not only the binding energy situation is different for this material class, but also the experimentally assessable activity curves look different, which was not rationalized yet.”
Therefore, identifying or tailoring new catalysts for specific reactions remains a hurdle. For instance, how can researchers efficiently determine how changing one element in the alloy will alter its properties? What is the optimal combination and how can this be easily determined?
Screening an infinite number of element combinations to produce the perfect catalyst is, of course, not a viable option. Instead, the team identified experimental parameters to help them identify if a specific alloy had potential activity using the oxygen reduction reaction as an initial platform. According to the study, the random assortment of five or more elements has a unique adsorption energy distribution — the adhesion of atoms, ions, or molecules onto a surface — pattern, which can help to predict specific electrochemical behavior.
“We elaborated how this will affect the shape of experimental response curves assessing the activity,” said the team. “Instead of one current increase as present for conventional catalysts, this curve is split up in at least 5 parts (for 5 elements), which we called ‘current waves’ and whose individual intensity is lower at first. By tailoring the elemental ratio of these elements, the intensity of those waves can be tailored accordingly. With this concept, an improved understanding of the working principles of this catalyst class is provided and a correlation between elemental constitution, the characteristic binding energy distribution and the shape of the activity response curves is achieved.”
“In order to reach very high [catalytic] activities, a good fit of the best adsorption peak maximum with the optimal binding energy as well as a high intensity of this peak corresponding to a high number of those sites is required,” stated the authors in their study.
Through their method, the group was able to identify two alloys (CrMnFeCoNiNb and CrMnFeCoNiMo) that were promising candidates for the oxygen reduction reaction, “whose composition can be optimized to complement the suitable position of the most active peak, and thus transfer the high activity to up-scaled applications.”
“The ultimate goal is to have a theoretical model at hand, which allows to calculate the best set of elements and their respective ratio for any reaction of choice,” said the authors. “We are currently collaborating with a computational model group to achieve this goal for the oxygen reduction reaction.
“However, every element combination has still to be tested individually and improvements in computational calculation time are required and the correct boundary conditions for each reaction have to be found. There are multiple aspects where a deeper investigation will be worthwhile, which combine multiple fields of theoretical computation, nanoparticle synthesis, material science, and electrocatalysis, and joining forces from experts in all fields presumably results in remarkable progress in this young, but highly promising field.”
In addition to enhancing researchers’ ability to screen for optimal combinations of elements, this study provides an important foundation for understand how this class of materials works and what properties and parameters are best used to help design new, enhanced materials.
Research article found at: T. Löffler, et al., Angewandte Chemie International Edition, 2020, doi.org/10.1002/ange.201914666
Updated February 10 to include quotes from the authors