In solid state physics, electrons or holes confined in 0-dimensional nanostructures, i.e., quantum dots, represent fruitful platforms for the realization of quantum-bits (qubits), exploiting spin and/or charge for quantum computation and simulation. However, qubits are inevitably coupled to the degrees of freedom of their surrounding environments, causing a loss of coherence that deeply affects qubit operations.
Great progress has been made for qubits hosted in semiconducting III-V compounds, such as GaAs, which provide advantages in terms of fabrication. However, materials belonging to group IV, such as Si and Ge, possess isotopes with zero nuclear spin, which allows them to reduce magnetic noise. Si qubits in particular have recently attracted much attention due to the immediate integrability with the existing Complementary Metal-Oxide Semiconductor (CMOS) technology found in the microelectronic industry.
In 2012, Zhan Shi and co-authors published a paper in which a new, three spin qubit is defined, resulting in what the team called a hybrid qubit. This new system owes its name to the advantageous combination of manipulation speed of a charge qubit with the longevity of a spin qubit.
This was achieved by confining three electrons in a double quantum dot by means of gate voltages and has since gained special interest in applications related to quantum computation due to advantages in fabrication, control, and electrical manipulation. The fluctuations of the global magnetic field do not affect the dynamics of the hybrid qubit, which is defined in a decoherence-free subspace. The main sources of decoherence comes from local magnetic fluctuations and charge fluctuations.
Research in recent years has been focused on ameliorating dominant sources of decoherence in order to increase the coherence time in a Si/SiGe hybrid qubit, and directly connect atomic scale disorder at the quantum well interface with the dephasing of the hybrid qubit.
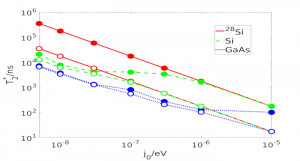
T2* as a function of j0 when σ E = 0 (28Si, red solid line), 3 neV (Si, green dashed line), and 100 neV (GaAs, blue dotted line). Two values of σ ε / ε 0 = 0.003 (filled marks) and σ ε / ε 0 = 0.03 (open marks) are studied.
Recently, Dr. Elena Ferraro and co-workers have proposed a theoretical study on the coherence time of the hybrid qubit, employing the quasi-static model in which both the magnetic and electrical disturbance are evaluated using disorder-averaged return probability.
Coherence time was evaluated through the number of coherent oscillations shown by the disordered return probability before it decays, and was extracted by adopting an envelope-fitting procedure. This work is based on the hypothesis that gate voltages are fixed and kept constant while the qubit evolves in time. With this approach, the coherence time of the hybrid qubit was extracted when model parameters take experimental values in the nuclear-free isotope 28Si, natural Si, and GaAs hosts.
Coherence time analysis, which is of great interest in view of its applications in quantum computation, was done with respect to a parameter (j0) that fully describes the qubit in terms of geometrical parameters and hosts material. A lower bound was found was found compared to literature values, and was beyond the scope of charge noise and the nature of the host materials, which did not affect the coherence times; decreasing (j0), the magnetic noise prevails. Moreover, the behavior of the quality factor, Q, was used to identify the best experimental conditions required to maximize the number of the hybrid qubit state oscillations, which is directly related to the gate operations.
“This research provides an in-depth theoretical study on the coherence time in semiconducting qubit and gives an important instrument for future works together with the development of experimental realizations,” says Dr. Ferraro.