Guest Editors Wee-Jun Ong, Zhiqun Lin and Kazunari Domen introduce a Special Issue of Particle & Particle Systems Characterization on “Artificial Photosynthesis: Mimicking Nature for Renewable Energy Production”. They discuss the utmost urgency of utilizing solar energy as a renewable energy source to substitute the exhaustible fossil fuels in the near future, which is a step closer to mimicking the ingenuity of nature (i.e. natural photosynthesis). Herein, the Special Issue focuses on important concepts in artificial photosynthesis for versatile energy- and environmental-related applications such as photo(electro)chemical H2 evolution, photoelectrochemical O2 evolution, photo(electro)catalytic CO2 reduction, pollutant degradation, and so forth.
In the 21st century, the widespread utilization of carbon-based fossil fuels (e.g. coal, oil and natural gas) for the generation of energy and electricity driven by rapid economic growth has come at a cost. Combusting 1 t of carbon in fossil fuels discharges beyond 3.5 t of carbon dioxide (CO2) and the accumulation of CO2 concentration has overwhelmingly surpassed 1 Tt in the atmosphere.[1] Astonishingly, the CO2 concentration in the atmosphere rose to above 410 ppm for the first time in April 2017 based on the Mauna Loa station, which has been continuously collecting and monitoring the atmospheric CO2 level.[2] The anthropogenic emission of CO2 at an alarming rate has thus resulted in global warming and climate change with unfavorable catastrophic effects.
According to the detailed 5th assessment report of the Intergovernmental Panel on Climate Change (IPCC) in 2014, it is emphasized that the global temperature rise should be limited to a maximal level of 2 °C to avert the severe impacts of global warming for the benefit of the people and ecosystems.[3] To attain this target, effective measures have been systematically taken by all parties, including academia and industry. Importantly, the long-term temperature goal has been recognized by the political arena, as revealed in the Paris agreement, which was signed on December 2015.[4]
To date, research in the arena of cutting-edge clean energy technologies has been thriving. Empowered by the rapidly-blossoming realm of nanoscience and nanotechnology, this has undoubtedly opened new frontiers in tailoring nanoparticles with novel functionalities for applications in renewable energy.[5] Among all renewable energy means, the utilization of solar energy, which is mimicked by the natural photosynthesis in green leaves, is a game-changing breakthrough in artificial photosynthesis to reduce the use of exhaustible fossil fuels in the long run.[6] Notably, natural photosynthesis converts approximately 1% of solar light energy into carbohydrates utilized by plants,[7] which can set a paradigm shift from an industry powered by fossil fuels to one powered by the sun.
Since the pioneering work on photocatalytic H2O splitting using TiO2 by Fujishima and Honda in 1972,[8] tremendous concerted efforts have been devoted to exploring high-efficiency light-responsive photocatalysts and photoelectrocatalysts for a broad range of energy- and environmental-related applications.[9] This includes water splitting to hydrogen and oxygen, reduction of CO2 to high-energy >C1 carbon-containing products, nitrogen fixation to ammonia, degradation of pollutants, selective organic transformation, and many more.[10] In light of that, photo(electro)chemistry has become the mainstream in this emerging frontier of science and technology at present. Based on the quick search for “photocatal*” and “photoelectro*” on the Web of Science (dated 30th November 2017), the number of publications has skyrocketed from ≈11,000 in 2010 to over 21,500 in 2017. As such, the burgeoning development and sustained production of advances in the booming field of solar-to-energy conversion have inspired us to put together a Special Issue specifically dedicated to “Artificial Photosynthesis: Mimicking Nature for Renewable Energy Production”. Figure 1 depicts the major topics in the present artificial photosynthesis research. The issue was conceived as a collection of selected contributions by researchers who are experts and/or rising stars in the forefront of this solar energy research platform. We are certain that these 15 peer-reviewed papers (comprising 1 Review, 3 Communications, and 11 Full Papers) will provide a clear mind map to the readers of Particle & Particle Systems Characterizations on the state-of-the-art research directions. In the following, a brief summary of the papers included in this Special Issue will be described to act as an overview for wide readerships.
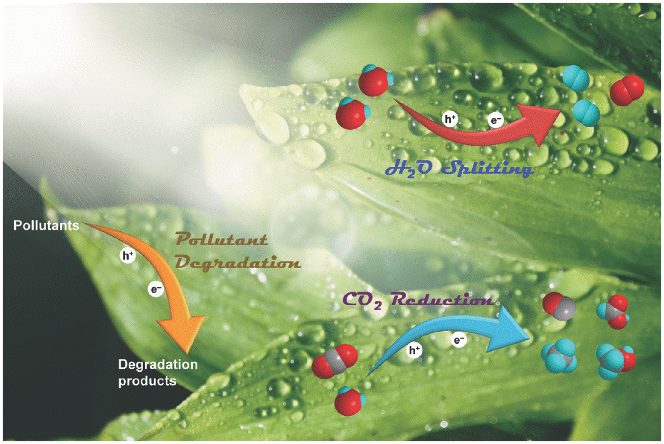
Figure 1. A selection of research topics covered in this Special Issue: photo(electro)chemical H2O splitting and CO2 reduction, and pollutant degradation.
One may naturally question, “Like living leaves, are there any efficient means to turn atmospheric CO2 into useful chemical fuels?” To answer the above question, recent progress of photoelectrocatalytic reduction of CO2 is reviewed by Lianzhou Wang and co-workers (article number 1700371). They summarize four typical configurations for the photoelectrocatalytic CO2 reduction, namely a photocathode coupled with an anode cell, a photoanode combined with a cathode cell, a photocathode integrated with a photoanode cell, and a photovoltaic-photoelectrocatalytic tandem cell, with an emphasis on the rational design of the electrodes to achieve high efficiency. In addition to photoelectrochemistry, Adam F. Lee and co-workers report the synthesis of delaminated CoAl-layered double hydroxide nanosheets (LDH-DS) combined with TiO2 nanotubes or nanoparticles for photocatalytic reduction of CO2 to CO without requiring sacrificial agents (article number 1700317). The photocatalytic enhancement is attributed to the improvement in photoinduced charge carrier separation as a result of the intimate formation of heterojunction interface between visible-light-active CoAl-LDH-DS and UV-triggered anatase TiO2. In another work, Junwang Tang and co-workers have fabricated two-dimensional (2D) BiVO4 nanoplates with large specific surface area coupled with nanocrystalline SnO2 for the photoconversion of CO2 and 2,4-dichlorophenol degradation (article number 1700320). Compared with bare BiVO4, the SnO2/BiVO4heterojunction demonstrates 7- and 4-fold increments for CO2 reduction to CH4 and degradation of 2,4-dichlorophenol, respectively, stemming from the role of built silicate bridges to facilitate electron transfer and migration.
In addition to CO2 reduction, light-driven water splitting to hydrogen and oxygen has garnered incessant research fascination lately. For the past several years, graphitic carbon nitride (g-C3N4) has become a vibrant field of research owing to its compelling band structures, visible-light-responsiveness, facile synthesis and Earth-abundant nature.[9a] Xiaobo Chen and co-workers investigate the effects of hydrogenation on g-C3N4nanosheets for photocatalytic hydrogen evolution and degradation of methylene blue (article number 1700038). In comparison with pristine g-C3N4, the remarkable enhancement of hydrogen production by 45% is ascribed to the synergistic effects brought by hydrogenation, including improved optical absorption, decreased band gap energy, enlarged specific surface area and increased donor density. In another related study, g-C3N4 subjected to consecutive annealing and ammonia treatments is reported by Hsisheng Teng and co-workers (article number 1700221). Upon ammonia treatment, it helps to repair the nitrogen vacancies of the g-C3N4 and also bridge the polymeric framework and melem oligomers. As a result, this promotes the charge separation and transport as well as introduces active amino sites for electron injection to evolve hydrogen in the presence of Pt, leading to a high apparent quantum yield (AQY) value of 17% at 420 nm.
Apart from that, Wee-Jun Ong and co-workers hybridize 2D g-C3N4 with one-dimensional (1D) Co2P nanorods by the means of a solution-phase technique under sonication to form 1D/2D Co2P/g-C3N4 heterojunction nanohybrids (article number 1700251). Benefitting from the natural sheet-like structure in g-C3N4 and the robustness of Co2P transition metal phosphide, the nanocomposite endows high visible-light photocatalytic hydrogen evolution without the assistance of Pt as the noble metal co-catalysts. Additionally, Kazuhiro Takanabe and co-workers present a molten-state synthesis method for the design of metal sulfide with single metals (Fe, Co, Ni, Cu and Zn) and quarternary CuGa2In3S8 using thiourea as a reactive precursor without capping agents (article number 1700183). Interestingly, the novel synthesis involves the formation of a variety of organic carbon nitride polymeric complexes around the metal sulfide nanoparticles. Taking the merit of heterointerface between quarternary CuGa2In3S8 and carbon nitride, the nanostructure exemplifies a high AQY value of 8.7% at 420 nm.
Besides the aforementioned g-C3N4 photocatalysts, Kazunari Domen and co-workers assess the physical properties and visible-light photocatalytic hydrogen evolution reaction of La5Ti2Cu(S1-xSex)5O7 (LTCS1-xSexO) (0 ≤ x ≤ 0.6) solid solutions developed by solid-state reactions at varying temperatures (article number 1700275). This work highlights the significance of regulating the particle size of narrow bandgap LTCS1-xSexO oxysulfoselenides so as to effectively harvest visible light for photocatalytic H2evolution. Jerome Claverie and co-workers develop a complex nanoarchitecture composed of TiO2 nanobelts, graphite-like carbon and Au nanoparticles (TiO2–C–Au hybrids) by employing an in situ surface polymeric encapsulation technique (article number 1700297). This method yields three different core@shell structures of TiO2–C–Au with dissimilar C and Au arrangements, giving rise to dramatically different photocatalytic and photoelectrocatalytic performances (e.g. hydrogen evolution, degradation of methylene blue and incident photo-to-current efficiency test). Guido Mul and co-workers present that methanol plays a predominant role on the kinetics of photodeposition of Pt on WO3 from H2PtCl6 solutions, as well as on the obtained morphology and oxidation state of the as-developed nanoparticles (article number 1700250). The authors claim that the extensive reduction of Pt in methanol is induced by methoxy-radicals, which have originated from the oxidation reaction of methanol with photogenerated holes.
Apart from the extensive works on photochemistry as mentioned above, a photoelectrochemical system involves the integration of a semiconducting material (photoabsorber) and a co-catalyst to facilitate charge transfer and separation in an electrochemical cell. There are two contributions focusing on the interfacial optimization between the photoactive materials and the co-catalyst. Li-Zhu Wu and co-workers demonstrate that the photoelectrochemical water splitting of CdSe quantum dots-sensitized photocathodes can be remarkably ameliorated by modulating the structural morphology of hole-transfer-layer of NiO toward a porous structure (article number 1700278). This confers an increase in the loading of quantum dots and simultaneously promotes the successful migration of holes, thus providing a viable approach to enhance the solar-to-fuel conversion efficiency of artificial photosynthesis. Hitherto, the majority of the research works on Si microwire arrays lies in the development of photocathodes for the photoelectrochemical hydrogen evolution. Hao Ming Chen and co-workers manifest a tunable electrodeposition technique for decorating isolated Ni nanoparticles as both protecting and oxygen evolution electrocatalyst onto the n-type Si microwire array photoanodes (article number 1700321). A highly stable photocurrent density of 10 mA cm−2 in 1 M KOH electrolyte can be attained for several hours without incorporating additional protecting layers other than Ni nanoparticles.
As we know, photochemistry and the related photoelectrocatalytic systems are not merely constrained to the production of hydrogen and oxygen from water splitting or the generation of carbonaceous products from the CO2 reduction. Zhiqun Lin and co-workers synthesize ternary sulfide Cu2Mo6S8 films with a needle-leaf-like architecture on the copper plates through a facile two-step route (article number 1700302). Owing to the larger surface area from the dendritic Cu2Mo6S8 structures and the inherently rapid charge transport of Cu2Mo6S8 compared to Cu(OH)2 nanowires, this renders a considerably high photocatalytic activity of the hierarchically-structured Cu2Mo6S8 films in the degradation of methylene blue. In another research, Kai A. I. Zhang and co-workers have designed a hybrid photocatalytic system comprising conjugated microporous polymer (CMP)-based organic semiconductors with immobilized TiO2nanoparticles for visible-light-triggered photocatalytic oxidative coupling reaction of amines and the selective oxidation of organic sulfides (article number 1700234). The high conversion and selectivity of the organic photoredox reactions are accredited to the presence of organic/inorganic heterojunction interface to boost the charge transfer and separation, which can serve as a robust photocatalyst to mediate other synthetic transformations. Aside from the pollutant degradation and selective organic transformations, Zhiyong Tang and co-workers have examined a series of commercially purchased semiconductor photocatalysts (e.g. ZnO, Fe2O3, CuO, Co3O4, TiO2, and WO3) for visible-light-driven asymmetric reactions with the aid of chiral ligands (article number 1700280). As such, this investigation will be a good guiding star for future semiconductor-based asymmetric photocatalysis.
In short, over the past decades, we have witnessed a significant renaissance in this pacey field of artificial photosynthesis, where great strides in nanostructure engineering are making headway toward the practical use in sustainable energy conversion and environmental remediation devices. As such, progress in this research arena has been so rapid that it is being considered for techno-economic viability, scale up and sustainability at the moment. In spite of the huge advancements in the smart design of nanomaterials for energy and environmental applications, it remains an up-hill task in the quest for cost-effective catalysts from ubiquitously Earth-abundant elements with robust activity, yield, stability and selectivity. Thus, the synergy cooperation from the researchers, scientists and industrial engineers across all disciplines of physics, chemistry, biology and engineering is mandatory to advance photo(electro)chemistry to the next new level. We are certain that the photo(electro)chemical approaches will achieve a technology readiness level in the next 10–20 years. To end this Editorial, we sincerely hope that the articles in this Special Issue of Particle & Particle Systems Characterizations will provide a clear overview to all readers on the diverse range of research subjects related to artificial photosynthesis. Let’s put in effort to jointly work together to make this world better! Last but not least, we are grateful to all the world-renowned scholars and reviewers for their huge contribution to this Special Issue. We also want to thank the editorial staff of Wiley-VCH, especially Mary De Vita, for their strong support and help with organizing this issue.
Wee-Jun Ong received his B.Eng and Ph.D. degrees in Chemical Engineering from Monash University. In 2015, he was a Visiting Research Fellow at the University of New South Wales and Monash University Clayton Campus, Australia. At present, he is a Research Scientist in IMRE, A*STAR in Singapore. His research interests focus on photocatalytic, photoelectrochemical and electrochemical water splitting, CO2 reduction, N2 fixation and H2O2 production for energy conversion and storage using semiconductor-based hybrid nanocomposites via experimental and density functional theory (DFT) studies. Currently, he serves as the Associate Editor of Frontiers in Chemistry and Frontiers in Materials, a Community Board Member of Materials Horizons, and an Editorial Board Member of Scientific Reports, Nanotechnology and Nano Futures. For more details, see: https://sites.google.com/site/wjongresearch/.
Zhiqun Lin is currently Professor of Materials Science and Engineering at the Georgia Institute of Technology. His research interests include polymer-based nanocomposites, block copolymers, polymer blends, conjugated polymers, quantum dots (rods, tetrapods and wires), functional nanocrystals (metallic, magnetic, semiconducting, ferroelectric, multiferroic, upconversion and thermoelectric) of different architectures (plain, core/shell, hollow and Janus), solar cells (perovskite solar cells, organic-inorganic hybrid solar cells and dye sensitized solar cells), batteries, hydrogen generation, hierarchically structured and assembled materials, and surface and interfacial properties. Currently, he serves as the Associate Editor of Journal of Materials Chemistry A, and an Editorial Advisory Board Member of Nanoscale.
Kazunari Domen received his B.Sc. (1976), M.Sc. (1979), and Ph.D. (1982) honors in chemistry from the University of Tokyo. He joined Chemical Resources Laboratory, Tokyo Institute of Technology, in 1982 as Assistant Professor and was promoted to Associate Professor in 1990 and Professor in 1996. In 2004, he moved to the University of Tokyo, Department of Chemical System Engineering as Professor. He has been working on overall water splitting reaction on heterogeneous photocatalysts to generate clean and recyclable hydrogen. Since 2000, he has been developing various kinds of photocatalysts for water splitting using visible light for efficient solar hydrogen production, with particular focus on surface chemical reaction dynamics. He is the President of Catalysis Society of Japan. Currently, he serves as the Associate Editor of Chemical Science, and the Chair of ChemPhotoChem.
[1] M. Mikkelsen, M. Jorgensen, F. C. Krebs, Energy Environ. Sci. 2010, 3, 43.
[2] Trends in Atmospheric Carbon Dioxide ( 2017); available from https://www.esrl.noaa.gov/gmd/ccgg/trends/weekly.html.
[3] Climate Change 2014 Synthesis Report: Contribution of Working Groups I, II and III to the Fifth Assessment Report of the Intergovernmental Panel on Climate Change (Eds: R. K. Pachauri, L. A. Meyer), IPCC, Geneva, Switzerland2014, p. 151.
[4] United Nations Framework Convention on Climate Change (UNFCCC) Paris Agreement ( 2015); available from http://unfccc.int/paris_agreement/items/9485.php.
[5] a) N. S. Lewis, D. G. Nocera, Proc. Natl. Acad. Sci. USA 2006, 103, 15729; b) I. Roger, M. A. Shipman, M. D. Symes, Nat. Rev. Chem. 2017, 1, 0003;c) W.-H. Wang, Y. Himeda, J. T. Muckerman, G. F. Manbeck, E. Fujita, Chem. Rev. 2015, 115, 12936;d) K. K. Sakimoto, N. Kornienko, P. Yang, Accounts Chem. Res. 2017, 50, 476; e) A. M. Beiler, G. F. Moore, Nat. Chem. 2017, https://doi.org/10.1038/nchem.2896.
[6] a) J. H. Montoya, L. C. Seitz, P. Chakthranont, A. Vojvodic, T. F. Jaramillo, J. K. Norskov, Nat. Mater. 2017, 16, 70; b) Y. Tachibana, L. Vayssieres, J. R. Durrant, Nat. Photon. 2012, 6, 511; c) K. Sivula, R. van de Krol, Nat. Rev. Mater. 2016, 1, 16010;d) J. Yang, D. Wang, H. Han, C. Li, Accounts Chem. Res. 2013, 46, 1900; e) D. Kim, K. K. Sakimoto, D. Hong, P. Yang, Angew. Chem. Int. Ed. 2015, 54, 3259.
[7] C. Liu, B. C. Colón, M. Ziesack, P. A. Silver, D. G. Nocera, Science 2016, 352, 1210.
[8] A. Fujishima, K. Honda, Nature 1972, 238, 37.
[9] a) W.-J. Ong, L.-L. Tan, Y. H. Ng, S.-T. Yong, S.-P. Chai, Chem. Rev. 2016, 116, 7159; b) X. Chen, N. Li, Z. Kong, W.-J. Ong, X. Zhao, Mater. Horiz. 2018, https://doi.org/10.1039/c7mh00557a;c) Y. Ma, X. Wang, Y. Jia, X. Chen, H. Han, C. Li, Chem. Rev. 2014, 114, 9987; d) C. Guo, J. Ran, A. Vasileff, S.-Z. Qiao, Energy Environ. Sci. 2017, https://doi.org/10.1039/c7ee02220d.
[10] a) P. Zhang, T. Wang, X. Chang, J. Gong, Accounts Chem. Res. 2016, 49, 911; b) K. Takanabe, ACS Catal. 2017, 7, 8006; c) T. Hisatomi, J. Kubota, K. Domen, Chem. Soc. Rev. 2014, 43, 7520; d) J. L. White, M. F. Baruch, J. E. PanderIII, Y. Hu, I. C. Fortmeyer, J. E. Park, T. Zhang, K. Liao, J. Gu, Y. Yan, T. W. Shaw, E. Abelev, A. B. Bocarsly, Chem. Rev. 2015, 115, 12888; e) S. J. A. Moniz, S. A. Shevlin, D. J. Martin, Z.-X. Guo, J. Tang, Energy Environ. Sci. 2015, 8, 731.